High-Frequency PCB Design Solutions
South-Electronic
South-Electronic is your ultimate source for high-quality, dependable, and highly valued high-frequency PCBs. Experience our comprehensive high-frequency PCB design service—from concept to completion, we guarantee accuracy, reliability, and strict adherence to industry standards.
在此添加您的标题文本
Why Choose South-Electronic?
- Customized High-Frequency Solutions
At South-Electronic, we specialize in customizing high-frequency PCBs to meet the specific demands of your project. Whether it‘s for telecommunications, aerospace, or consumer electronics, our designs ensure optimal signal integrity and minimal loss, perfectly aligning with your technical requirements.
- Advanced Design Capabilities
Our design team is experienced in creating both simple and complex multi-layer configurations. We focus on precision engineering and effective functionality to design high-frequency PCBs that exceed your expectations and propel your projects to success.
- Cutting-Edge Manufacturing Techniques
We use the latest in advanced manufacturing technology to make high-quality, complex PCBs that meet the strictest standards. Our precision manufacturing process makes sure that every PCB we build performs and lasts.
- End-to-End Service
We’re with you every step of the way, from the initial consultation and design to material selection, prototyping, and manufacturing, to quality assurance and on-time delivery. Our four-step process ensures complete support and customer satisfaction.
- Competitive Advantage through Innovation
Our high-frequency PCBs are engineered to enhance your product performance, giving you a competitive edge in the market. By optimizing your devices for high-speed and high-frequency applications, we help make sure your products stand out.
- Quality and Customer Satisfaction:
We're ISO9001 certified and committed to the highest standards of quality and customer service. We offer flexible ordering options, competitive pricing, and a guarantee of satisfaction, making us a trusted partner in your electronic manufacturing needs.
Related Project We had Done
Customer Reviews
Common Questions
Most Popular Questions
High-frequency PCBs are designed to transmit signals at very high speeds with minimal loss, which is crucial for applications in telecommunications, aerospace, and advanced computing. They ensure reliable and efficient performance in systems that require rapid signal processing.
Yes, South-Electronic specializes in customizing high-frequency PCB designs to meet the specific requirements of your projects. We tailor everything from material selection to layout design to ensure your PCB perfectly suits your needs.
We use a variety of advanced materials specifically suited for high-frequency applications, including FR-4, Polyimide, PTFE (Teflon), and others that provide enhanced signal integrity and thermal stability.
The lead time can vary depending on the complexity of the project and customer requirements. However, typical projects move from consultation to delivery within 3-6 weeks.
The lead time can vary depending on the complexity of the project and customer requirements. However, typical projects move from consultation to delivery within 3-6 weeks.The lead time can vary depending on the complexity of the project and customer requirements. However, typical projects move from consultation to delivery within 3-6 weeks.
Our high-frequency PCBs are used across a range of industries including telecommunications, consumer electronics, aerospace, and healthcare, particularly where high-speed and reliable performance are essential.
Yes, South-Electronic supports both small and large volume orders to accommodate all types of projects, ensuring flexibility and scalability for our customers.
Designing high-frequency PCBs involves challenges like managing signal integrity and minimizing interference. We address these issues through advanced engineering techniques, using high-quality materials, and precision in layout design.
Yes, we offer comprehensive prototyping services to test and refine your high-frequency PCB designs. This ensures the final product meets your technical requirements and performance expectations.
South-Electronic is ISO 9001 certified, UL, ISO14001, CE , ROHS,IATF16949, ISO13485 ensuring that our manufacturing processes meet the highest industry standards for quality and reliability.
Send us a message
The more detailed you fill out, the faster we can move to the next step.
在此添加您的标题文本
أبجد هوز حطي كلمن سعفص قرشت ثخذ ضظغ
The Complete Guide for High Frequency PCB Design
Cahpter 1
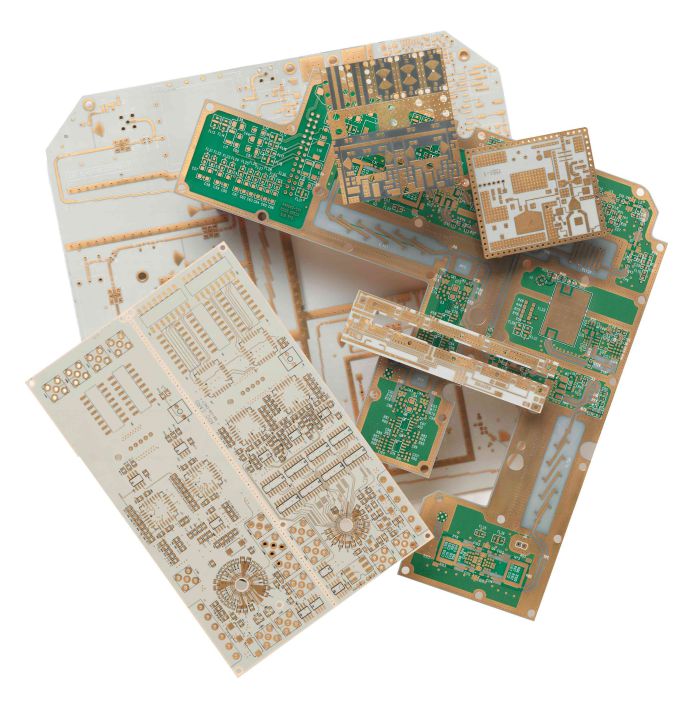
Introduction to High-Frequency PCBs
Are you ready to delve into the world of high-frequency PCBs and discover their critical role in modern electronics? High-frequency PCBs are specialized circuit boards designed for handling electromagnetic signals that operate at frequencies above 1 GHz. This capability makes them essential for applications that require fast signal processing without significant losses, ensuring both efficiency and precision in performance.
What are High-Frequency PCBs?
High-frequency PCBs are not just any circuit boards; they are engineered to excel in environments where quick and reliable signal transmission is paramount. These boards are typically made with materials that have low dielectric loss, such as FR-4 or polytetrafluoroethylene (PTFE), which help maintain signal integrity even at high speeds. This intrinsic property allows them to handle the rapid on-off switching of digital signals and the seamless transmission of analog signals across various electronic devices.
Why Are High-Frequency PCBs So Important?
In today’s technology-driven world, the importance of high-frequency PCBs cannot be overstated. They are the backbone of many applications that you rely on every day. From your smartphone’s WiFi and GPS systems to more complex systems like radar and satellite communications, these PCBs ensure that devices operate with the accuracy and speed required in a high-stakes digital age.
Moreover, as the demand for faster and more reliable electronic devices continues to grow, the role of high-frequency PCBs becomes even more pivotal. They are key to innovation in telecommunications, defense, aerospace, and consumer electronics, where they contribute to advancements in technology that push the boundaries of what is possible.
By choosing high-frequency PCBs, you are not just opting for superior electronic performance; you are also investing in the future of technology. These PCBs stand at the forefront of modern electronics, driving progress in countless industries by enabling faster, more reliable, and more efficient electronic communication. Whether you’re developing cutting-edge consumer electronics or sophisticated aerospace systems, high-frequency PCBs offer the capabilities you need to succeed in today’s fast-paced technological landscape.
Cahpter 2
Materials and Substrates for High-Frequency PCBs
When you’re diving into the realm of high-frequency PCB design, the choice of materials and substrates becomes critical. The performance of your PCBs at high frequencies hinges on these foundational components. Selecting the right materials is not just about ensuring functionality; it’s about maximizing efficiency, signal integrity, and the overall performance of your electronic devices.
What Materials are Used in High-Frequency PCBs?
High-frequency PCBs require materials that have exceptional electrical properties to handle the fast signal propagation without significant losses. Here are some of the most commonly used materials in high-frequency PCBs:
PTFE (Polytetrafluoroethylene): Widely known under brand names like Teflon®, PTFE is renowned for its low dielectric constant and low dissipation factor, which are crucial for minimal signal loss at high frequencies. It’s the go-to material for applications that require superior microwave performance.
FR-4: While standard FR-4 is a common material for lower frequency applications, high-performance FR-4 grades are available that offer improved dielectric properties for moderately high-frequency applications. This makes FR-4 a cost-effective option when extreme performance is not required.
Rogers Materials: These substrates are specifically designed for high-frequency PCBs. Rogers materials such as RO4003C and RO4350B provide lower dielectric losses, better impedance control, and thermal performance, making them ideal for demanding applications in aerospace, telecommunications, and automotive industries.
Ceramic Filled Laminates: These substrates incorporate ceramic fillers to improve thermal conductivity and reduce thermal expansion, which is vital for maintaining the structural integrity and performance consistency of PCBs at high frequencies.
How Do These Materials Affect Signal Integrity and Performance?
The choice of substrate material directly impacts the signal integrity of your high-frequency PCBs in several ways:
Dielectric Constant (Dk): A lower dielectric constant helps in reducing signal delay. Materials like PTFE have a lower Dk, which is preferable for high-frequency signal transmission as it allows faster signal speed with less impedance variability.
Dissipation Factor: Also known as the loss tangent, this factor measures how much of the electromagnetic energy is lost due to the inherent properties of the substrate material. Materials with lower dissipation factors, like Rogers and PTFE, are essential in minimizing energy losses in high-frequency applications.
Thermal Management: High-frequency operations can generate significant heat. Materials like ceramic filled laminates are crucial because they dissipate heat more effectively, preserving the integrity of the circuit and its performance over time.
Dimensional Stability: Materials such as Rogers and ceramic-filled laminates exhibit superior dimensional stability under thermal stress, which is crucial for maintaining tight tolerances in high-frequency circuits.
Choosing the right materials for your high-frequency PCBs isn’t just about meeting the minimum requirements; it’s about enhancing the performance and reliability of your final product. Each material brings its own set of properties that can either amplify or hinder the efficacy of your high-frequency PCB designs, influencing everything from signal speed and clarity to thermal management and mechanical stability. Therefore, understanding and selecting the optimal substrate is a pivotal decision that can define the success of your high-frequency electronic applications
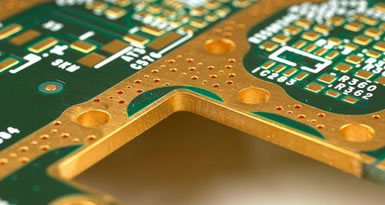
Cahpter 3

Design Considerations for High-Frequency PCBs
Designing high-frequency PCBs is an art that combines advanced engineering with precision execution. When you step into the world of high-frequency PCB design, every decision you make from the layout to the material choice impacts the performance and reliability of the final product. Let’s delve into the key design factors and techniques that are pivotal for optimizing high-frequency applications.
Key Design Factors for High-Frequency PCBs
Material Selection: As we’ve discussed, the choice of material is foundational. For high-frequency applications, you need materials with low dielectric constants and low loss tangents to enhance signal integrity. Materials such as PTFE (Teflon), Rogers, and ceramic-filled laminates should be at the top of your list. These materials not only support higher frequencies but also minimize signal losses, providing a robust base for your designs.
Impedance Control: In high-frequency PCBs, controlling impedance is crucial to prevent signal reflections and transmission losses. This involves precise calculations and adjustments to the width and spacing of the traces, depending on the dielectric properties of the substrate. Achieving consistent impedance requires meticulous design practices and often collaboration with material suppliers to ensure specifications are met.
Trace Geometry: The shape and layout of the traces significantly affect performance. Use smooth, curved traces instead of angular ones to minimize signal reflection and crosstalk. Also, the trace thickness should be optimized to balance between resistance, inductance, and capacitance, crucial for maintaining signal integrity at high frequencies.
Techniques to Minimize Signal Loss and Improve Reliability
Use of Ground Planes: Implementing solid ground planes below the signal layers can significantly reduce electromagnetic interference (EMI) and provide a stable reference voltage level, which is essential for maintaining signal integrity. Ground planes also help in heat dissipation, adding to the overall reliability of the PCB.
Shielding and Guard Traces: To further protect against EMI and external noise, consider using shielding techniques. Metal shielding can be incorporated over sensitive areas of the PCB. Additionally, guard traces with vias connected to the ground can be used around high-frequency traces to isolate them from other components of the circuit.
Thermal Management: High frequencies can lead to increased thermal loads, so effective thermal management is crucial. Design PCBs with thermal relief pads and heat sinks if necessary. Also, selecting materials with good thermal conductivity can help dissipate heat more efficiently, preserving the integrity and longevity of the circuit.
Via Design: In high-frequency PCBs, the design of vias plays a significant role. Use blind and buried vias to reduce the path of signal transmission, thus minimizing signal degradation. The placement and size of vias should be carefully planned to avoid impedance discontinuities.
Component Placement: Strategic placement of components is essential to minimize path lengths for critical signals, thus reducing opportunities for signal loss and interference. Components should be arranged to optimize the signal flow and minimize crossing high-frequency paths, which can introduce noise and loss.
Testing and Prototyping: Always prototype your designs before full production. Testing your prototypes allows you to identify and rectify issues related to signal integrity and functionality. This step is crucial for ensuring that your design not only meets the desired specifications but also performs reliably in real-world applications.
By carefully considering these design factors and employing these techniques, you can significantly enhance the performance and reliability of your high-frequency PCBs. Each decision in the design process is a step towards achieving a product that not only meets but exceeds the rigorous demands of high-frequency applications.
Cahpter 4
Signal Integrity and Management
When you’re designing high-frequency PCBs, you’re dealing with the challenge of maintaining signal integrity. Signal integrity is the lifeblood of your electronic devices. It’s what makes sure the signals you’re sending across your PCB aren’t distorted or disrupted. As you move into higher frequencies, signal integrity becomes even more important and more difficult to manage. Let’s talk about some of the challenges and best practices for maintaining signal integrity so your devices work the way they’re supposed to.
Challenges in Maintaining Signal Integrity at High Frequencies
Signal Attenuation: As frequency increases, signal loss becomes more pronounced due to the inherent properties of the materials and the PCB design. This can result in weaker signals at the receiver end, affecting the overall performance of your electronic device.
Reflection and Crosstalk: Improper impedance matching and closely packed traces can lead to signal reflection and crosstalk between channels. These phenomena not only degrade the quality of the signal but can also cause data corruption and loss of information.
Electromagnetic Interference (EMI): High-frequency circuits are more susceptible to external and internal noise sources, which can induce unwanted signals and disrupt normal operations. Managing EMI is crucial to maintain the integrity of your high-frequency signals.
Solutions and Best Practices for Managing Signal Integrity
Careful Material Selection: Choosing the right substrate material is your first line of defense in ensuring signal integrity. Materials with low dielectric constants and low dissipation factors, such as PTFE or Rogers substrates, are ideal as they minimize signal loss and support higher speed signal transmission.
Impedance Control: Maintaining uniform impedance throughout your PCB is crucial. This involves designing trace geometries and using controlled dielectric materials to match the impedance of the source and load. Use impedance calculators and simulation software to model and tweak your designs for optimal performance.
Strategic Trace Routing: Minimize crosstalk and reflection by routing critical high-frequency signals on separate layers or using shielded traces. Employ differential signaling where possible, as it helps to cancel out noise and improves signal integrity.
Utilizing Ground Planes: Incorporate solid and continuous ground planes in your PCB design. Ground planes reduce loop area and provide a return path for current, significantly mitigating the risk of EMI and signal reflection.
Decoupling and Filtering: Place decoupling capacitors close to power pins of active components to stabilize power supply fluctuations and reduce noise. Additionally, use filtering techniques to eliminate unwanted high-frequency components from your signals.
Advanced Testing and Simulation: Leverage advanced simulation tools to predict and analyze signal behavior before physical prototyping. Simulations can help identify potential issues like EMI effects, impedance mismatches, and signal reflections, allowing you to make necessary adjustments early in the design process.
Regular Reviews and Iterative Design: Adopt a practice of regular design reviews and iterative improvements. Evaluating your design at different stages enables you to identify and rectify issues related to signal integrity, enhancing the reliability and performance of the final product.
By understanding these challenges and implementing these best practices, you can significantly improve the signal integrity of your high-frequency PCB designs. Remember, every design decision you make—from material selection to trace routing—affects how well your product performs in real-world applications. Ensuring pristine signal integrity is not just about meeting technical specifications; it’s about delivering a product that stands out for its reliability and superior performance.
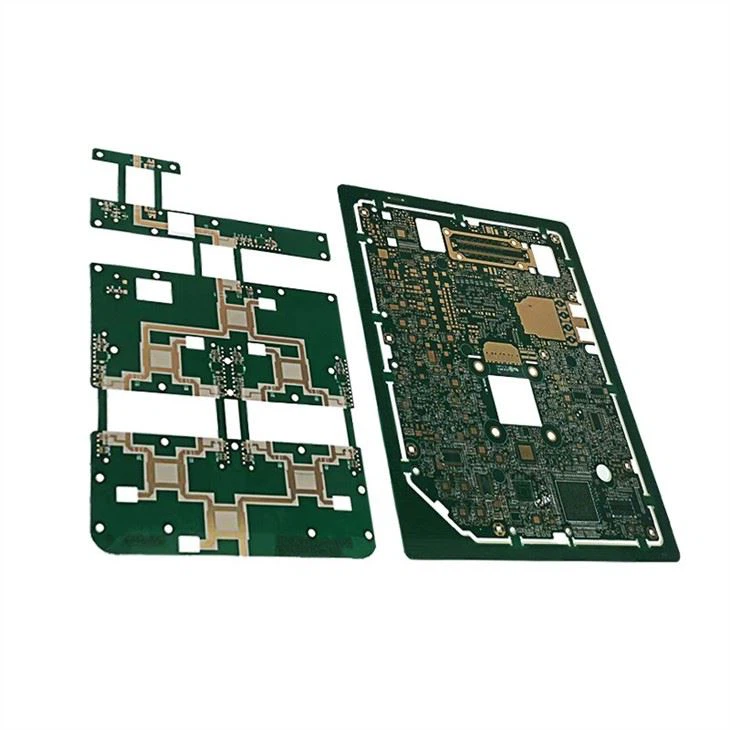
Cahpter 5
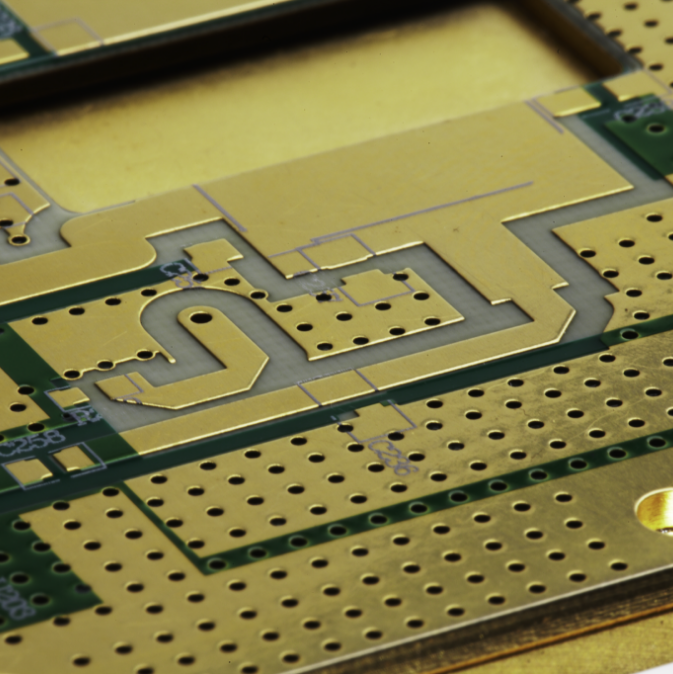
Layer Stackup and Configuration
When you dive into the world of high-frequency PCB design, understanding the strategic importance of layer stackup and configuration becomes paramount. The way you stack and configure the layers of your PCB not only influences the manufacturing process but also significantly impacts the performance of the final product. Let’s explore how effective layer stackup configurations can optimize your high-frequency PCBs and the benefits they bring to the table.
Why is Layer Stackup Important?
Layer stackup refers to the arrangement of copper and insulating layers in a PCB. In high-frequency applications, this configuration is critical because it affects everything from signal integrity and impedance control to thermal management and electromagnetic interference (EMI) shielding. An optimized layer stackup ensures that high-frequency signals travel with minimal loss and interference, making it a cornerstone of successful PCB design.
Exploring Effective Layer Stackup Configurations
Standard Multilayer Stackup: This common configuration involves alternating signal and ground planes. For high-frequency PCBs, ensuring that signal layers are closely coupled to ground layers minimizes signal loop areas and reduces the risk of EMI. This setup helps in maintaining cleaner signal transmission and improving overall electromagnetic compatibility (EMC).
Symmetrical Stackup: In high-frequency designs, a symmetrical stackup can be particularly beneficial. This configuration involves mirroring the layer arrangement around a central core, which helps in balancing the overall PCB structure, reducing warping during manufacturing, and maintaining consistent electrical performance across the board.
High-Density Interconnect (HDI) Stackup: HDI technology involves the use of finer spaces and lines, smaller vias, and denser component placement. For high-frequency applications, HDI stackups can significantly reduce signal path lengths, improving signal integrity and reducing cross-talk. Moreover, HDI allows for more compact designs, essential in modern, space-constrained electronic devices.
Benefits of Various Stackup Methods
Improved Impedance Control: With carefully planned layer stackups, you can achieve precise impedance control, which is vital for high-frequency signal transmission. By configuring the thickness and spacing of the layers appropriately, you can maintain consistent impedance levels across the PCB, reducing signal reflections and losses.
Enhanced Signal Integrity: Effective layer stackups reduce the likelihood of crosstalk and signal attenuation. By segregating high-speed signal layers from potential noise sources and using ground planes as shields, you ensure that your signals remain robust and clear over their transmission paths.
Better Thermal Management: Stackup can influence how heat is distributed and dissipated across the PCB. By strategically placing thermal layers or heat-sink layers, you can manage the heat generated by high-frequency operations, thus protecting sensitive components and enhancing the reliability of the board.
Increased Durability and Reliability: A well-thought-out layer stackup contributes to the physical stability of the PCB. It helps in managing mechanical stresses and reduces the likelihood of structural failures, such as delamination or cracking, which are critical in high-frequency applications where material integrity is paramount.
Optimized Real Estate: Especially in HDI designs, effective stackup configurations allow you to make the most of the available PCB real estate. This is crucial when you need to pack more functionality into smaller devices, a common requirement in today’s high-tech applications.
By mastering the art of layer stackup and configuration, you not only enhance the performance and reliability of your high-frequency PCBs but also pave the way for innovations that meet the demands of tomorrow’s technology landscape. Remember, each layer in your stackup plays a critical role, and understanding how to configure them effectively can be the key to unlocking the full potential of your high-frequency designs.
Cahpter 6
Impedance Control and Matching
When you venture into the realm of high-frequency PCB design, one of the most critical aspects to master is impedance control and matching. This isn’t just a technical necessity—it’s the linchpin that ensures your PCB performs optimally, preventing signal degradation and ensuring reliable communication between components. Let’s dive into why impedance control is so crucial and how you can achieve and maintain perfect impedance matching across your PCB.
Why is Impedance Control Crucial in High-Frequency PCBs?
Impedance control is essential because it directly affects the integrity and performance of the signals traveling through your PCB. In high-frequency applications, any mismatch in impedance can lead to signal reflections, standing waves, and loss of signal integrity, which can degrade the performance of your entire system. Effective impedance control ensures that the electrical signals propagate smoothly without disruptions, providing consistent performance and minimizing signal loss.
Methods to Achieve and Maintain Impedance Matching
Material Selection: The journey to perfect impedance control starts with the right material selection. You need substrates with consistent dielectric properties to ensure uniform impedance levels across the board. Materials like FR-4, Rogers, and Teflon are popular choices due to their stable dielectric constants and loss tangents, which are critical for maintaining impedance integrity at high frequencies.
Trace Geometry Design: The dimensions and layout of your PCB traces play a pivotal role in impedance control. To match impedance, you must carefully design trace width, thickness, and spacing according to the dielectric properties of your substrate and the target impedance value. Tools like impedance calculators and field-solving software can help you model and optimize these parameters effectively.
Use of Ground and Power Planes: Implementing continuous ground and power planes beneath the signal layers can significantly improve impedance stability. These planes not only provide a solid reference point for the signals but also help in reducing electromagnetic interference and crosstalk, further stabilizing the impedance.
Stackup Configuration: An optimized layer stackup can aid in maintaining consistent impedance across the PCB. Ensure that signal layers are closely coupled with ground planes and that the stackup is symmetric to avoid any variations in the impedance due to physical and thermal stresses during PCB fabrication and operation.
Controlled Impedance Routing: When routing high-frequency traces, maintain consistent impedance by avoiding abrupt changes in trace width or direction, which can cause impedance discontinuities. Techniques such as using curved traces instead of angles and maintaining uniform trace separations can help in preserving the integrity of the impedance.
Termination Techniques: Proper termination of traces is crucial for impedance matching, especially where high-frequency signals are concerned. Use techniques like series or parallel termination to match the impedance at the ends of transmission lines, minimizing reflections and ensuring smooth signal transmission.
Regular Testing and Verification: Throughout the PCB design and manufacturing process, regularly test and verify the impedance using TDR (Time Domain Reflectometry) and other measurement techniques. This ensures that the impedance remains within specified limits and that any issues are identified and corrected before final production.
By diligently applying these methods, you ensure that every component on your PCB communicates effectively, free from the pitfalls of impedance mismatch. This not only boosts the performance of your high-frequency PCB but also enhances the reliability and longevity of your electronic device. Remember, in the world of high-frequency electronics, precision in impedance control isn’t just an option—it’s a necessity for staying competitive and achieving technological excellence.
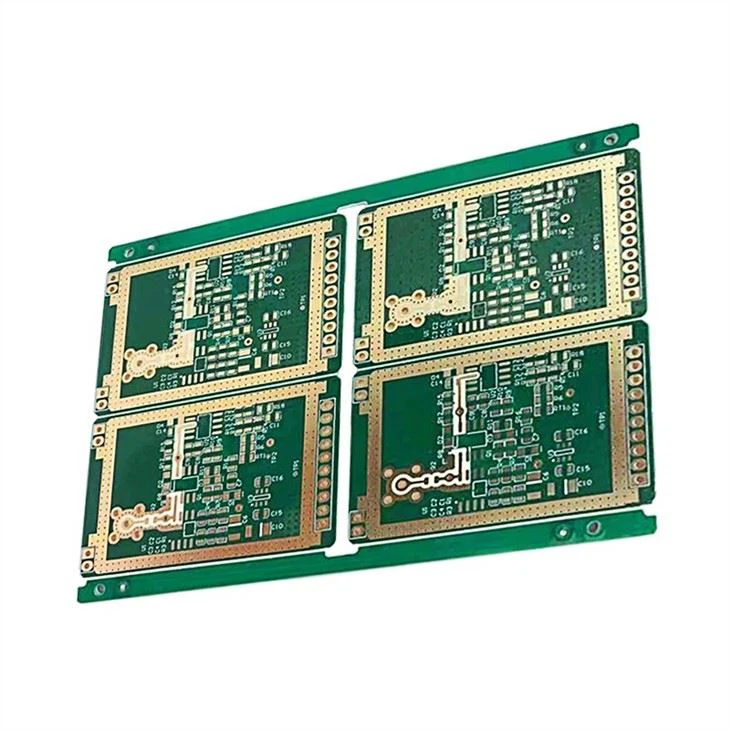
Cahpter 7
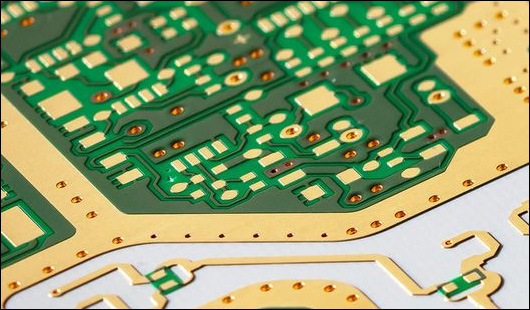
Thermal Management in High-Frequency PCBs
When you get into high-frequency PCB design, managing the heat becomes a big deal. As the frequencies go up, the power density goes up, and that means the temperature goes up. That can affect the reliability and performance of your electronic devices. So, let’s talk about the challenges and how you can deal with them so that your high-frequency PCBs will work the way that you need them to.
Challenges of Managing Heat in High-Frequency PCBs
Increased Power Density: High-frequency operations often require components that consume more power, leading to increased heat generation per unit area. This can create hot spots on the PCB, which are detrimental to both the electronic components and the substrate.
Component Sensitivity: Many high-frequency components are sensitive to temperature changes. Excessive heat can lead to accelerated aging, reduced performance, and even failure of these components, compromising the overall functionality of the device.
Material Limitations: The substrates and materials used in high-frequency PCBs, such as PTFE or high-performance laminates, have specific thermal characteristics. Some materials may not dissipate heat as efficiently as needed, which can lead to temperature build-up and increased thermal stress.
Compact Layouts: As device sizes shrink and the demand for more compact electronic products increases, the packing density of components on PCBs grows. This denser layout further complicates the effective dissipation of heat.
Strategies for Effective Thermal Management
Material Selection: Start with selecting the right substrate materials that not only meet your electrical requirements but also have good thermal conductivity. Materials like metal-core PCBs (MCPCBs), which include a metal layer (commonly aluminum or copper) beneath the thermal pads of critical components, can help significantly in heat dissipation.
Thermal Vias: Implement thermal vias to efficiently transfer heat from the top layer where components are mounted to lower layers or to the opposite side of the PCB, which can then dissipate heat more effectively. These vias act as small heat pipes, spreading the heat over a larger area to reduce hot spots.
Heat Sinks and Cooling Mechanisms: Attach heat sinks directly to heat-generating components, or use onboard cooling mechanisms such as fans or liquid cooling systems. These are especially useful in high-power applications where passive cooling might not be sufficient.
Component Placement and Layout Optimization: Carefully plan the placement of components to minimize thermal coupling and avoid creating hot spots. Allow sufficient spacing between high-power components to enable adequate air circulation and heat dissipation. Use simulation tools during the design phase to model thermal performance and adjust layouts as necessary.
Thermal Relief Pads: Use thermal relief pads to help manage the connection of heat-generating components to the ground or power planes. These pads can prevent excessive heat transfer to other parts of the PCB that might be sensitive to temperature changes.
Regular Thermal Testing: Conduct thermal testing during the prototype and manufacturing phases to understand how your PCB performs under operational conditions. Use infrared cameras, thermocouples, and other temperature-sensing devices to identify hot spots and thermal gradients, and refine your design based on these findings.
By integrating these strategies into your PCB design process, you can effectively manage the thermal challenges posed by high-frequency operations. Good thermal management not only enhances the durability and reliability of your high-frequency PCBs but also ensures that your devices function within safe temperature limits, preserving the longevity and performance of every component on the board. Remember, effective thermal management is not just about keeping components cool; it’s about ensuring that your entire system works harmoniously at optimal temperatures.
Cahpter 8
Testing and Prototyping for High-Frequency PCB
When you’re developing high-frequency PCBs, the phases of testing and prototyping are not merely steps in the process—they are your assurance of quality and reliability before full-scale production. These stages allow you to identify potential issues and optimize your design, ensuring that your PCBs meet both your standards and those of the industry. Let’s delve into the essential tests and prototyping methods that are crucial for high-frequency PCBs, guiding you through how to make these steps a cornerstone of your design process.
Essential Tests for High-Frequency PCBs
Time Domain Reflectometry (TDR) Testing: This test is essential for measuring the impedance characteristics of your PCB. TDR testing helps you detect any impedance discontinuities which can cause signal reflections, crucial for maintaining signal integrity at high frequencies.
Signal Integrity Tests: Perform tests to analyze the quality of the signals on your PCB. These include measuring parameters such as rise and fall times, jitter, and eye diagrams, ensuring that the signals are strong and clear without interference, even at high frequencies.
Electromagnetic Compatibility (EMC) Testing: To ensure your high-frequency PCB does not emit unwanted electromagnetic interference (EMI) or is susceptible to external EMI, EMC testing is critical. This helps you verify that your PCB complies with international EMC standards, crucial for market acceptance.
Thermal Testing: Since high-frequency operations can generate significant heat, conducting thermal tests using thermal imaging cameras or sensors is essential. This ensures that your thermal management strategies are effective and that your PCB can operate reliably under various temperature conditions.
Power Integrity Testing: Assess the power distribution network (PDN) performance on your PCB to ensure that all components receive stable and clean power without excessive noise or voltage drops, critical for the reliable operation of high-frequency components.
Prototyping Methods and Their Importance
Rapid Prototyping: Utilize techniques like CNC machining to quickly produce PCB prototypes. This allows you to iterate designs rapidly based on testing feedback, significantly speeding up the development process.
3D Printing of PCBs: For very complex or unconventional designs, 3D printing can be used to prototype PCBs, allowing for greater flexibility in design and the ability to test form factors that are difficult to create with traditional methods.
Low-Volume Fabrication: Before moving to mass production, producing a small batch of PCBs can be invaluable. This low-volume run can be used for extensive functional testing and to gather feedback from potential users or field tests.
Why Prototyping and Testing are Vital
Risk Reduction: Early and iterative prototyping combined with rigorous testing significantly reduces the risk of failures in full-scale production. It helps identify and rectify design flaws and ensures the final product meets required specifications.
Cost Efficiency: Identifying issues in the prototype phase is much less costly than making changes during full-scale production or, worse, recalling faulty products from the market.
Performance Optimization: Testing and prototyping give you the opportunity to fine-tune the performance of your PCBs, ensuring that they operate at peak efficiency and are optimized for their intended application.
Stakeholder Confidence: Demonstrating thoroughly tested and prototyped PCBs builds confidence among stakeholders, including investors, clients, and regulatory bodies, that the product is reliable and ready for market.
By integrating comprehensive testing and robust prototyping into your development process, you ensure that your high-frequency PCBs are not just functional but are fine-tuned machines ready to meet the challenges of the tech-intensive environments in which they will operate. Remember, these steps are your best tools to mitigate risks and perfect your products before they hit the market.

Cahpter 9
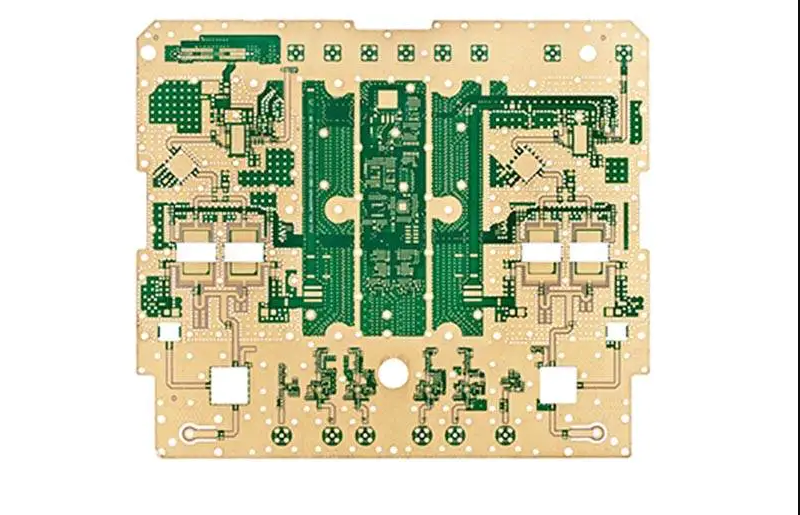
Manufacturing Processes for High-Frequency PCBs
When you dive into the manufacturing of high-frequency PCBs, you are entering a realm where precision and adherence to quality standards are not just goals, they are necessities. Every step in the manufacturing process plays a critical role in determining the performance and reliability of your final product. Understanding the nuances of these processes and how different techniques affect the outcome is crucial. In this post, we will explore the sophisticated world of high-frequency PCB manufacturing, guiding you through each step and its impact on the final PCB.
Overview of the Manufacturing Process for High-Frequency PCBs
Material Selection: The journey starts with choosing the right materials. For high-frequency PCBs, materials like PTFE, Rogers, and ceramic-filled laminates are preferred due to their low dielectric constants and loss tangents, which are essential for minimizing signal loss and ensuring signal integrity at high frequencies.
Circuit Pattern Printing: The design of your high-frequency PCB is transferred onto the chosen substrate using advanced printing techniques. This can involve photolithography, where a photoresist is applied and exposed to light to create the circuit pattern, or direct imaging techniques for more complex or finer designs.
Etching: After printing the circuit pattern, the unwanted copper is removed through an etching process. Precision in this step is crucial; any deviation can affect the impedance and performance of high-frequency signals. Controlled etching techniques are used to ensure that only the unwanted copper is dissolved, leaving a clean and precise circuit pattern.
Layer Stacking and Lamination: Multiple layers of the PCB are stacked and laminated under heat and pressure. For high-frequency PCBs, ensuring that each layer aligns perfectly is critical for maintaining the integrity of the electrical pathways and for effective impedance control. Any misalignment can lead to signal integrity issues and reduced performance.
Drilling and Plating: Holes or vias are drilled to establish connections between different layers of the PCB. For high-frequency applications, the precision of via placement and the quality of the plating inside the vias are paramount. These vias must be perfectly aligned and have smooth copper plating to ensure reliable electrical connections and to maintain signal integrity.
Solder Mask Application: A solder mask is applied over the copper traces to protect them from oxidation and to prevent solder bridges during component placement. For high-frequency PCBs, the solder mask must be applied uniformly to avoid any unintended capacitance or insulation issues.
Surface Finishing: The PCB receives a surface finish that serves as a protective coating and provides a reliable solderable surface for mounting components. Options like ENIG (Electroless Nickel Immersion Gold) or HASL (Hot Air Solder Leveling) are chosen based on their impact on signal integrity and their compatibility with high-frequency applications.
Testing and Quality Control: Every high-frequency PCB undergoes rigorous testing, including impedance testing, signal integrity tests, and functionality checks. These tests ensure that each PCB meets the stringent requirements necessary for high-frequency operations.
Impact of Different Manufacturing Techniques on the Final Product
Advanced Material Handling: Using specialized materials like PTFE requires adjustments in the standard PCB manufacturing processes, such as modified etching and lamination techniques to handle these sensitive materials without damaging their properties.
Precision Engineering: The requirement for high precision in etching, drilling, and layer alignment is heightened in high-frequency PCB manufacturing. Slight deviations can significantly impact performance, making precision engineering a cornerstone of the process.
Specialized Finishing Techniques: The choice of surface finish has a more pronounced effect on high-frequency PCBs, as some finishes can introduce unwanted inductance or capacitance. Selecting the right finish is crucial for maintaining optimal signal performance.
By mastering these manufacturing processes and understanding their implications, you ensure that your high-frequency PCBs not only function as intended but also stand up to the demands of modern electronic applications. Each step, from material selection to final testing, is geared towards achieving the highest quality and performance, ensuring that your PCBs deliver where it matters most.
Cahpter 10
Future Trends in High-Frequency PCB Design
As we look towards the horizon of high-frequency PCB design, it’s clear that the field is poised for transformative changes. Emerging technologies and materials are setting the stage for revolutionary advancements that will reshape how we design and use PCBs in high-frequency applications. Let’s explore what these trends entail and predict how they might influence the future landscape of PCB technology.
Emerging Technologies and Materials
Liquid Crystal Polymer (LCP) Substrates: Liquid Crystal Polymer is gaining attention as a substrate material for high-frequency PCBs due to its excellent electrical properties, including low dielectric constant and loss tangent, which are essential for minimal signal loss and maximum signal integrity. LCP is also highly resistant to moisture and has superior thermal stability, making it ideal for extreme environments.
Integrated Passive Devices (IPDs): The integration of passive components directly onto the PCB substrate is a growing trend. IPDs help reduce the size and weight of PCB assemblies, enhance signal integrity by minimizing interconnections, and improve reliability by reducing the number of solder joints. This technology is particularly beneficial in high-frequency applications like telecommunications and aerospace.
3D Printed Electronics: Additive manufacturing or 3D printing is set to revolutionize PCB manufacturing by allowing more complex geometries and multi-dimensional structures. This technology enables the integration of electronic functionality into the very fabric of components, opening new avenues for device miniaturization and complex high-frequency applications.
Flexible and Stretchable PCBs: As the demand for wearable technology and flexible electronics grows, so does the need for PCBs that can conform and stretch. New materials and fabrication techniques are being developed to produce high-frequency flexible PCBs that can maintain performance under mechanical stress and deformation.
Predictions on How These Advancements Will Shape the Future
Greater Integration and Miniaturization: The future of high-frequency PCB design is likely to see even more compact and integrated solutions. Technologies like IPDs and 3D printing will enable designers to embed more functionality into smaller areas, pushing the limits of miniaturization while improving performance and reliability.
Enhanced Performance in Harsh Environments: With materials like LCP, future high-frequency PCBs will be more durable and capable of operating under extreme environmental conditions. This will be crucial for expanding the use of high-frequency electronics in sectors such as aerospace, military, and automotive industries.
Wider Adoption of Flexible and Stretchable PCBs: The ongoing development of flexible substrates will lead to broader adoption in consumer electronics, medical devices, and even in emerging fields like soft robotics. These PCBs will not only need to perform at high frequencies but also withstand bending and stretching, challenging designers to think beyond traditional design paradigms.
Sustainability in PCB Manufacturing: As environmental concerns become more pressing, the PCB industry will likely shift towards more sustainable manufacturing processes. This could include the use of bio-based materials, recyclable substrates, and energy-efficient fabrication techniques.
Advanced Testing and Simulation Tools: With the complexity of high-frequency PCBs increasing, advanced simulation and testing tools will become even more crucial. These tools will need to predict and mitigate issues related to electromagnetic interference, thermal management, and mechanical stress long before physical prototypes are built.
By staying ahead of these trends, you’ll not only be preparing for the future of high-frequency PCB design but also shaping it. Each advancement brings us closer to more powerful, efficient, and versatile electronic devices, heralding a new era of technology that transcends today’s limitations
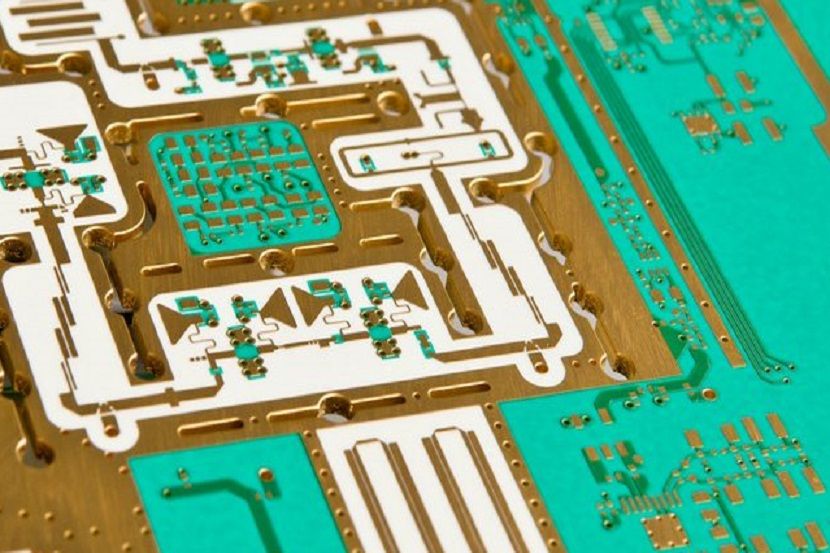
Get in touch
Where Are We?
Industrial Park, No. 438 Donghuan Road, No. 438, Shajing Donghuan Road, Bao'an District, Shenzhen, Guangdong, China
Floor 4, Zhihui Creative Building, No.2005 Xihuan Road, Shajing, Baoan District, Shenzhen, China
ROOM A1-13,FLOOR 3,YEE LIM INDUSTRIAL CENTRE 2-28 KWAI LOK STREET, KWAI CHUNG HK
service@southelectronicpcb.com
Phone : +86 400 878 3488
Send us a message
The more detailed you fill out, the faster we can move to the next step.
